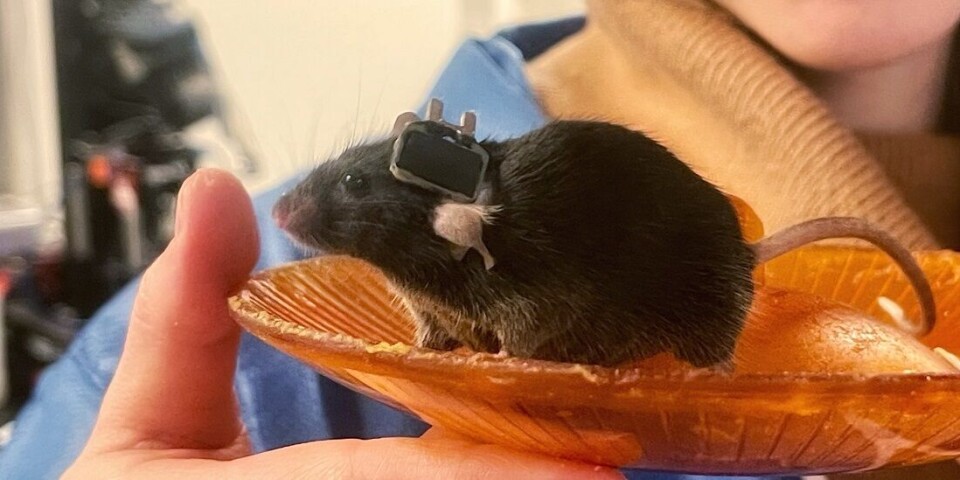
Breakthrough discoveries of how the brain stores memories
How do you remember what happened yesterday, or in your childhood, or right now? Norwegian researchers are on the trail of a new and important piece in the large puzzle that is our brain.
Imagine that your brain was unable to remember things in order, as if every memory was just a snapshot, randomly stored in the brain.
"The ability to organise elements into sequences is a fundamental biological function essential for our survival," the Kavli Institute writes in a press release.
Most viewed
If memories were not stored in order, we would not be able to communicate, find our way to places, or remember what we are doing right now. The world would cease to be a place that makes sense.
But how does the brain manage to store memories in the correct order?
Researchers at NTNU’s Kavli Institute believe they have found the answer, recently published in the journal Nature.
“I believe we have found one of the brain's prototypes for building sequences,” Professor Edvard Moser says in the press release.
Video clips, not snapshots
Thinking of memories as snapshots is not a faithful description, according to Edvard Moser. He suggests that we think of memories as videos.
“All your experiences in the world extend over time. One thing happens, then another thing, then a third," Professor May-Britt Moser states in the press release.
But researchers who study activity in the brain often study just short moments. There is a mismatch between what is typically studied, namely milliseconds, and the time the brain typically spends on performing tasks, somewhere between ten seconds and up to several minutes, according to Soledad Gonzalo Cogno from the Kavli Institute. She is the lead researcher behind the new study.
The aim of the mouse experiment led by Cogno was to find out how the brain organises experiences in specific sequences, so that we can navigate and remember things in a functioning way.
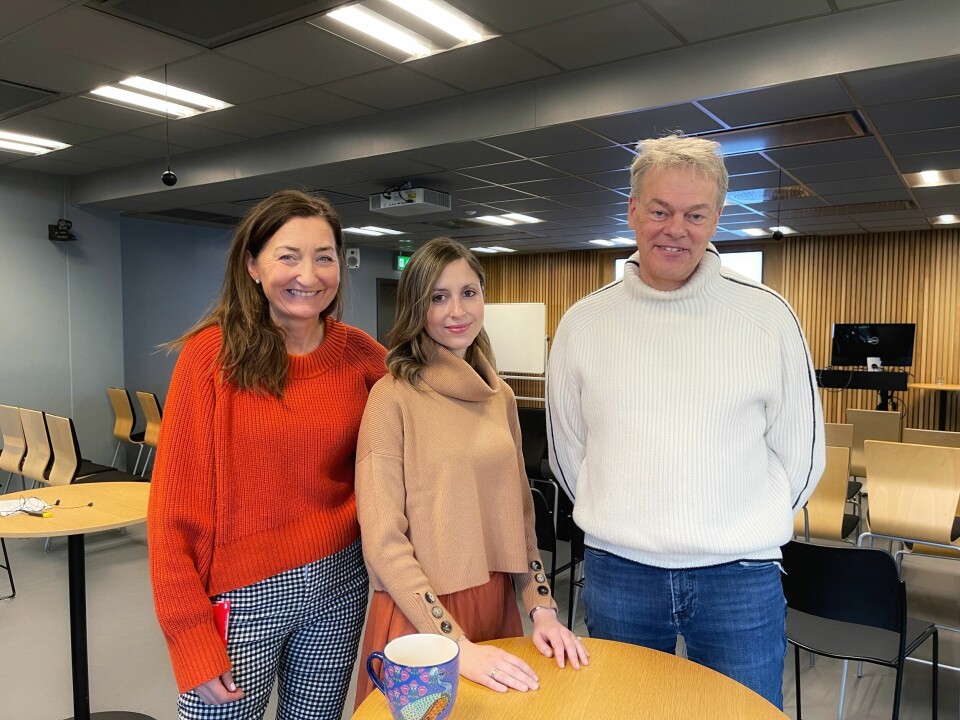
Mice in the dark
The Kavli researchers conducted an experiment with mice to find out how the nerve cells, or neurons, talk to each other in the slower rhythm at which many of the brain's functions operate.
But our brain processes huge amounts of information at any given time, so how do we capture these slow memories? To minimise the amount of sensory noise, the mice in the study were placed on a running wheel in a cage that was completely dark. There were no tasks for them to perform, and no reward they could achieve. They could choose whether they wanted to run or rest.
And while the mice had free rein in the dark, the researchers measured what was happening in the medial entorhinal cortex of the mouse brain. This region of the brain, besides being central in the formation of memories, is also responsible for our sense of place.
This is where the Mosers had previously discovered grid cells, often referred to as the brain's GPS in popular science, for which they received the Nobel Prize with John O'Keefe in 2014.
What the researchers found in the mouse brain was a pattern of nerve cells that moved in a sort of coordinated wave, "like rhythms in a symphony," according to the press release. The wave moved slowly. It took two minutes for it to travel through the neural network before starting over again.
Even more exciting, according to the researchers, was that while the nerve cells surged forward, they also organised themselves into ordered sequences.
This finding constitutes the prototype for how the brain stores episodic memory, i.e., the events you remember from life.
Does the pattern change with age?
“This is a breakthrough,” confirms Clive Bramham, a professor from the University of Bergen.
“The findings indicate something fundamental about brain functions related to memory that hasn’t been shown before,” he says.
In addition to studying what happened in the centre for sense of place and memory, the Kavli researchers also looked at two other areas of the brain. However, they did not find the same wave-like repetitions of activity there.
“What they observed is a firing pattern that appears in this particular area, of cells that follow in rhythm and come again and again. It's something that is intrinsic to the brain, as they write in the article. It may be that this already exists in the brain in the foetal stage,” Bramham says.
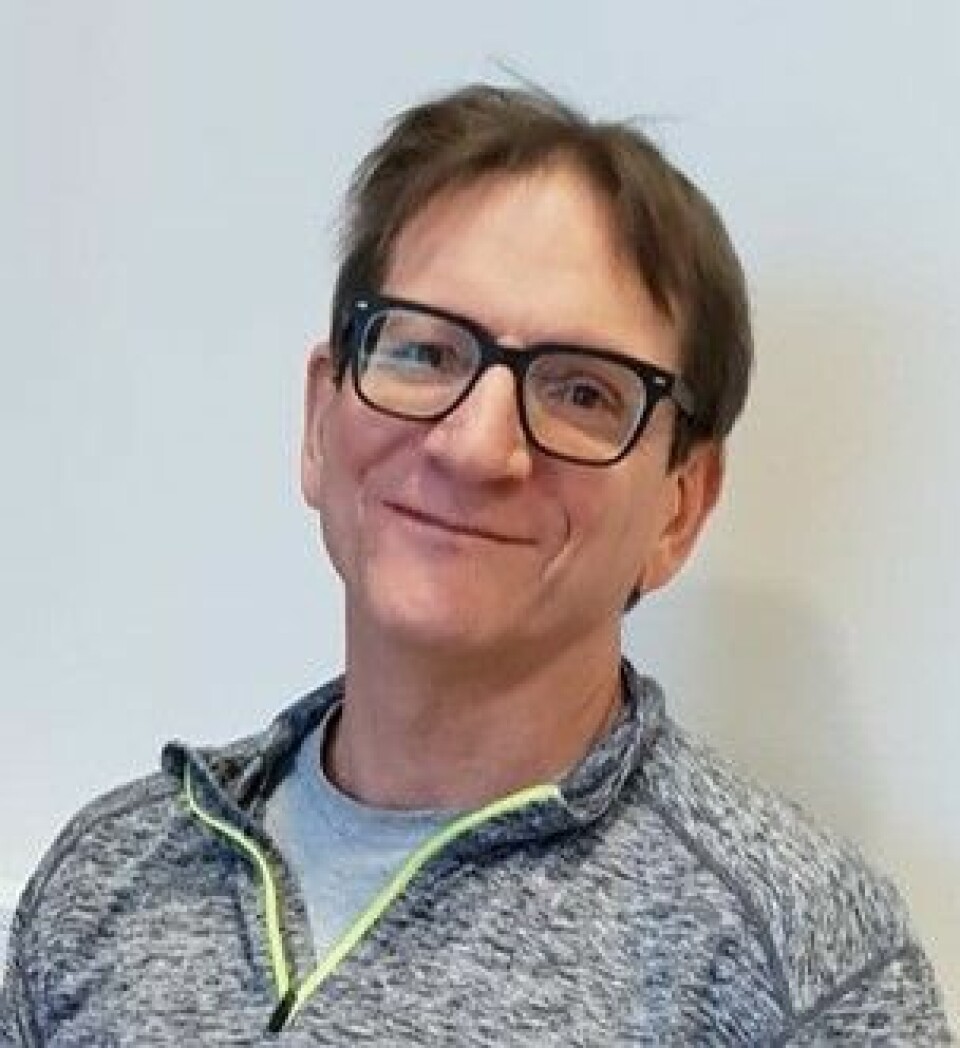
All memory is linked to a sequence. Until now, there has been no good explanation for how these sequences work.
“So this discovery forms a framework to begin to understand it. The exciting next step is to look at how these wave sequences change, such as with behaviour and age,” he says.
What does this pattern look like in someone with Alzheimer's, for example? Could it be relevant for how we treat dementia with medications?
“It will be important to map whether these fluctuations are actually important for navigation and memory, and how they are affected by diseases like Alzheimer's dementia,” he says.
Mice and humans
At the Bramham laboratory, Clive Bramham researches the plasticity of the brain. He and his colleagues study tiny components in the brain cells to find out how memory works, whereas the Kavli team researches how these cells form networks.
In the long run, Bramham believes it would be exciting to connect the two fields and bridge the gap between the study of single-cell functions such as gene regulation and network functions.
He finds the actual study from the Kavli Institute, and not just the results, very impressive.
“This work is a tour de force in terms of technology alone. It's the technology that makes such a study possible, so that researchers can record data from hundreds and thousands of cells and measure their activity. This is a technically challenging study to perform,” he says.
In one of the studies cited in the new research article, researchers have found something similar in the human brain. But the recently published Nature study involves mouse brains. To what extent does the research also apply to the human brain?
“Mice and rats can navigate, they remember, they have an entorhinal cortex and so do we. There are similar structures in our brains. So it's not a stretch to believe that these fundamentals could be very similar. It’s interesting to see if there are differences, but the findings are transferable at a high level,” Bramham says.
Many new questions to answer
Eric Hanse is a professor of neurophysiology at the University of Gothenburg.
“This is truly exciting work and completely new to me,” says Hanse, who is a researcher in the same field.
“You can probably call it a breakthrough, because I haven’t seen this type of very slow and wave-like nerve cell activity in the awake state before. The article does provide some references to similar phenomena from previous studies,” he says.
Hanse notes that the study points to many new questions, which is typical of such breakthroughs.
What are the mechanisms behind these slow waves? Do we only see them in the entorhinal cortex? What kind of relationship do they have with more well-known and fast waves like theta and gamma waves?
Edvard Moser tells NRK (link in Norwegian) that the mechanism came as a bit of a surprise to them.
“What we found was different than what we imagined it would be, but now it makes sense,” he says.
This finding is significant, the professor says, but we won't know exactly how significant it is compared to other findings until a number of years from now.
“But it's a new phenomenon that opens the door for more research that can provide answers to how the brain works,” he says.
———
Translated by Ingrid P. Nuse
Read the Norwegian version of this article at forskning.no